端粒和端粒酶的作用
端粒(Telomere)是20世纪30年代被发现的位于染色体3’末端的DNA-蛋白复合物[6]。人类的端粒序列是一段重复的碱基序列(TTAGGG),并由shelterin蛋白包绕[7,8]。端粒序列在人出生时最长,出生后由于后随链半保留复制和氧化损伤等因素,导致染色体端粒在细胞复制后进行性缩短[9-11]。当细胞复制次数达到约50次时(Hayflick极限),染色体由于端粒结构缩短而形成末端融合,并进一步导致细胞衰老、细胞周期阻滞和凋亡等[12,13]。此外,端粒缩短还会通过激活p53-p21通路、ATM和ATR信号通路,诱导双链断裂DNA损伤反应(DDR),并最终导致细胞死亡[14-16]。因此,缩短的端粒作为细胞增殖的屏障,是防止细胞永生化和肿瘤形成的关键机制[17,18]。
端粒酶是唯一一种利用线性染色体的3’端作为引物,合成端粒DNA的逆转录酶[19,20]。端粒酶的关键成分包括其催化亚基端粒酶逆转录酶(TERT)和作为逆转录模板的端粒酶非编码RNA成分(TERC)(图1)[21,22]。TERC在正常细胞和肿瘤细胞中均广泛表达,作为端粒酶活性主要限速步骤的TERT在约90%的肿瘤细胞中高表达,而在绝大多数正常细胞中不表达[23-25]。因此,TERT的表达可被视为肿瘤的标志物[26]。除了延长端粒外,TERT还在肿瘤血管生成、形成炎性和免疫抑制肿瘤微环境等方面发挥着重要作用[27-30]。
图1. 端粒和端粒酶复合物结构示意图。Shelterin蛋白复合物锚定于端粒上,以稳定端粒结构;端粒酶复合物利用催化亚基TERT和自身的RNA模板(TERC),从染色体3’端合成端粒。
TERT启动子突变
TERT启动子突变主要发生在5号染色体TERT转录起始位点上游-124bp和-146bp两个热点处(chr5: 1, 295, 228 and 1, 295, 250),两种突变均为胞嘧啶-胸腺嘧啶(C>T)突变(即C228T和C250T)[25],这两种突变导致TERT的表达量上调2-4倍[37,38]。C228T和 C250T突变为转录因子GABPA结合提供了新的结合位点,从而上调TERT的表达(图2)[39]。脑膜瘤中TERT启动子突变相对罕见(5~20%)[34,40]。
图2. A. TERT启动子C228T和C250T突变能形成新的ETS结合基序,转录因子ETS家族成员GABPA和GABPB形成异源四聚体,并结合在ETS位点上,上调TERT表达。B. 人类不同实体瘤中TERT突变的发生率。
图3. TERT启动子突变的脑膜瘤患者,总生存期(A)和无进展生存期(B)均更短。
TERT启动子甲基化


参考文献
作者简介
“阔然生物医药科技(上海)有限公司(Shanghai KR Pharmtech, Inc. , Ltd.)”简称“阔然基因”成立于2015年,总部位于上海,旗下拥有三家高新技术企业和一家专精特新小巨人企业,致力于提供多应用场景的一站式分子诊断解决方案,包括癌症早期筛查、诊断与监测以及药物研发服务,拥有6款IVD产品注册申报储备,服务中国500余家医院和科研机构,建立了庞大的基因组数据库。阔然拥有上海和徐州双研发中心,两家医学检验实验室(“零缺陷”通过CAP权威认证)和精准医学科技研究院,凭借“产品+服务”模式开展肿瘤分子诊断、肿瘤免疫微环境检测等业务,为临床医生对患者的诊疗提供一体化解决方案,实现“精准医疗践行者”的理念,推动我国医疗事业发展。
阔然精准诊疗脑肿瘤产品CaptioX-M是一款以NGS技术为基础的检测产品。CaptioX-M用于脑膜瘤的分子诊断,涵盖了脑膜瘤复发相关的67个基因(NF2、TERTp、CDKN2A/B、ARID1A和BIP1等)、预后相关的染色体(22q、1p、5、10等)拷贝数变异和潜在靶向治疗相关基因的信息,脑膜瘤CaptioX-M可用于脑膜瘤患者分子分型、预后评估和辅助医生制定治疗策略。
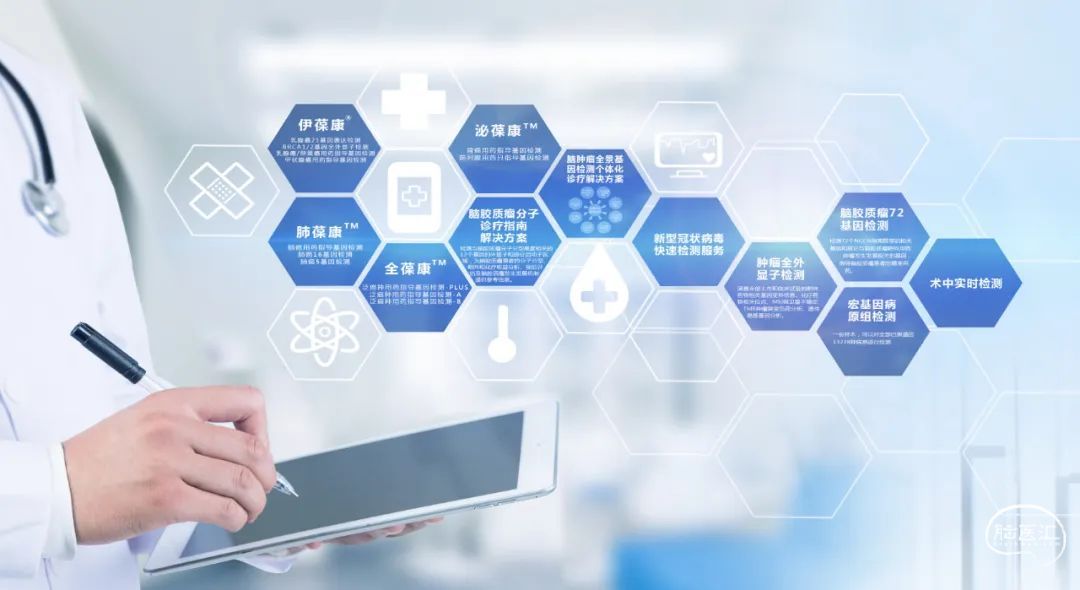